Hydrogel Skins: Soft Interfaces for Intelligent Systems
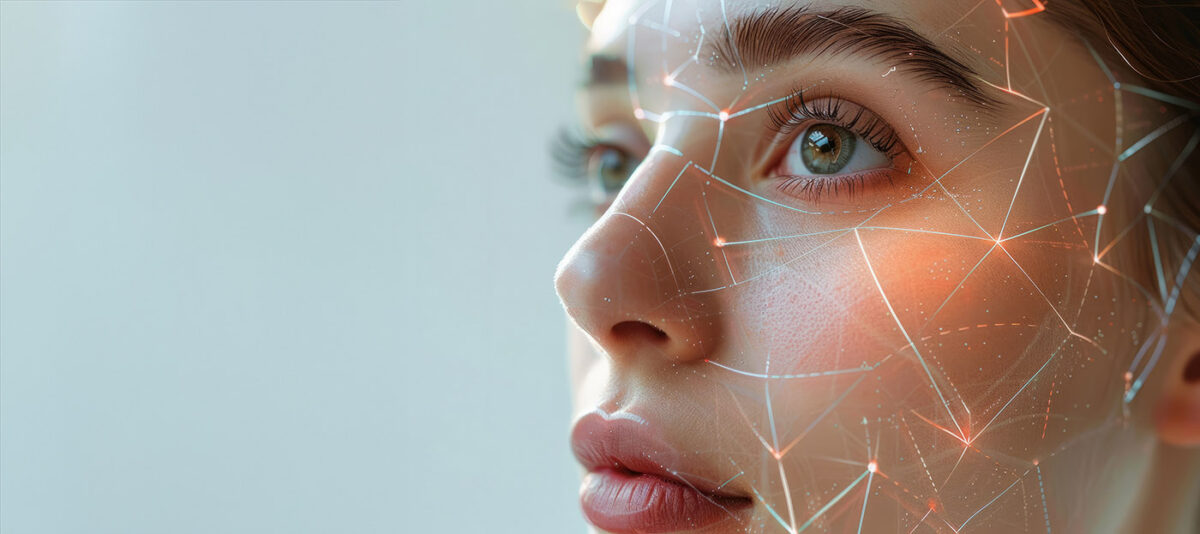
Hydrogels, composed of 3D polymer networks with high water content, closely mimic the mechanical properties of human tissues and organs, making them ideal for contact with human skin.
Hydrogel-based self-powered artificial skins are employed in various Human Machine Interface (HMI) applications, such as touch panels, gesture recognition, and health monitoring. Moreover, these skins enable real-time monitoring of physiological parameters, including heart rate, blood pressure, and muscle tension. Additionally, attaching them to robots enhances their ability to sense touch and interact with their environment.
You can also read: Innovative Hydrogel Coatings for Medical Catheters
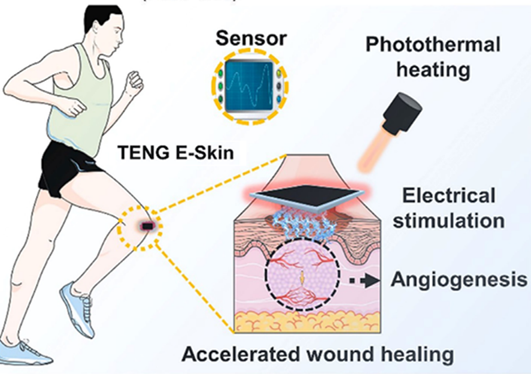
Hydrogel-based electronic skin patches. Courtesy of Advanced Intelligent System.
Researchers use diverse types of hydrogels for self-powered artificial skins, including ionic hydrogels, ionic-liquid hydrogels, metal-based hydrogels, carbon-based hydrogels, MXene-based hydrogels, and conductive polymer-based hydrogels.
Each type offers unique properties and advantages. For instance, ionic hydrogels provide high sensitivity and self-powered capabilities, while metal-based hydrogels offer superior conductivity and mechanical strength.
Power Mechanisms of Hydrogels
Hydrogel-based artificial skins can generate power through various mechanisms, including triboelectric, piezoelectric, and thermoelectric effects. The triboelectric effect generates electrical energy through friction between different materials, while the piezoelectric effect converts mechanical stress into electrical signals. The thermoelectric effect harnesses temperature gradients to produce electrical power.
- Triboelectric Effect: This effect uses friction and contact between materials to generate electrical charges. Hydrogel-based TENGs (triboelectric nanogenerators) convert mechanical energy into electricity for tactile sensing and motion monitoring.
- Piezoelectric Effect: This effect converts mechanical stress into voltage via the deformation of piezoelectric materials. These sensors detect physiological signals for neuromodulation and muscle excitation applications, such as pulses and vocal cord movements.
- Thermoelectric Effect: Includes thermodiffusional ions and thermogalvanic effects. These mechanisms create electrical potential from temperature gradients, allowing for the conversion of body heat into usable energy. Wearable sensors and energy-harvesting devices use this effect.
Illustration of the demands of hydrogel-based self-powered artificial skins for human-machine interfaces. Courtesy of Advanced Intelligent System.
Combining Transparency, Stretchability, and Multifunctionality for Next-Generation Interfaces
Hydrogel-based self-powered artificial skins mark a significant leap in human-machine interfaces (HMIs). These materials combine transparency, stretchability, high self-powered performance, self-healability, frost resistance, and multifunctionality, making them ideal for intelligent control, medical care, and touch screens.
- Self-Healability: Self-healing hydrogels restore functionality after damage, ensuring long-term durability.
- Frost Resistance: Antifreeze agents like lithium chloride in hydrogels enable operation in subzero temperatures.
- Transparency: Hydrogels like polyacrylamide (PAAm) with lithium salts offer up to 98% transparency, enabling clear touch panels and gesture recognition systems.
- Stretchability: Hydrogels, such as polyampholytic-clay nanocomposite-based hydrogels, can achieve fracture strains exceeding 1500%, thus providing excellent flexibility and resilience. Indeed, this property is essential for creating artificial skins that can conform to complex surfaces and withstand repeated mechanical deformation.
- Multifunctionality: Hydrogel-based skins sense stimuli, generate energy, and provide tactile feedback, thereby enhancing human interaction with intelligent systems. For example, Yu et al. created a multifunctional bionic skin using a highly stretchable hydrogel with carbon quantum dots, which exhibited high sensitivity, wide sensing range, and excellent cyclic stability. Consequently, this multifunctionality makes hydrogel-based artificial skins versatile tools for advanced HMIs, further enhancing the interaction between humans and intelligent systems.
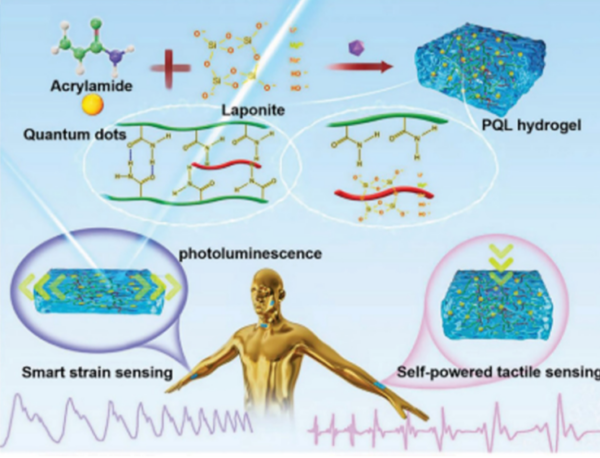
Multifunctional self-powered electronic skin. Courtesy of Advanced Intelligent System.
Future For Hydrogel-based Artificial Skin
Despite their advantages, hydrogel-based artificial skins face challenges such as susceptibility to freezing, dehydration, and mechanical damage. Researchers are addressing these issues by developing hydrogels with enhanced frost resistance, dehydration tolerance, and self-healing properties. Researchers have designed antifreeze hydrogels to maintain performance in extreme temperatures, ensuring the reliable operation of artificial skins in various environments.
Future research aims to improve the durability, functionality, and scalability of hydrogel-based self-powered artificial skins. Innovations in materials science and engineering will lead to even more advanced HMIs, further integrating human intelligence and abilities into intelligent machines, and revolutionizing the way humans interact with technology.